Ultrathin Polarization Insensitive Multiband Metamaterial Microwave Absorber for C, X and Ku Band Applications
DOI:
https://doi.org/10.5281/zenodo.10984018Keywords:
metamaterial absorber, multiband, ultrathin, microwave absorptionAbstract
A polarization independent multiband metamaterial microwave absorber is designed and characterized. The absorber design comprise a 0.03 mm thick copper metallic pattern arranged in fourfold symmetric manner to complete one unit cell. Each metallic pattern is designed using multiple strip resonators of different lengths connected together. The top layer is separated by 1.56 mm thick FR4 dielectric from back annealed 0.03mm thick continuous copper plate. The simulated responses derived using HFSS shows that the intended absorber exhibits five absorption peaks at 5.9 GHz, 7.8 GHz, 10.9 GHz, 17 GHz and 18 GHz. with an absorption of 92.5%, 99%, 83%, 90% and 91% respectively. The electrical dimensions of the unit cell are 0.314λ × 0.314λ computed at 5.9 GHz. The designed structure is polarization independent due to four-fold symmetric design configuration and therefore exhibit the same absorption response for both TE and TM polarizations for normal incident of the EM wave. The absorption response for oblique incidence angles has also been examined for both polarizations and the absorber exhibit above 80% absorption at oblique angles of incidence. The multiband absorption make it a potential candidate for various RF applications such as RCS reduction, EMI shielding etc.
Downloads
References
R.S. Kshetrimayum. (2004). A brief intro to metamaterials. IEEE Potentials, 23(5), 44–46.
D. Schurig, J. J. Mock, B. J. Justice, S. A. Cummer, J. B. Pendry, A. F. Starr, & D. R. Smith. (2006). Metamaterial electromagnetic cloak at microwave frequencies. Science, 314, 977–980.
M. A. Antoniades, & G. V. Eleftheriades. (2003). Compact linear lead/lag metamaterial phase shifters for broadband applications. IEEE Antennas and Wireless Propagation Letters, 2, pp. 103-106.
M. Gil, J. Bonache, & F. Martín. (2008). Metamaterial filters: A review, 2, 186-197.
Fang, N., H. Lee, C. Sun, & X. Zhang. (2005). Sub-diffraction-limited optical imaging with a silver superlens. Science, 308, 534–537.
A. Sanada, C. Caloz, & T. Itoh. (2004). Characterization of the composite right/left handed transmission lines. IEEE Microwave Wireless Components Letters, 14, 280–282.
R.W. Ziolkowski. (2006). Metamaterial-based efficient magnetically small antennas. IEEE Transactions on Antennas and Propagation, 54(7).
N. I. Landy, S. Sajuyigbe, J. J. Mock, D. R. Smith, & W. J. Padilla. (2008). Perfect metamaterial absorber. Phys. Rev. Letters, 100, 207402.
Y. I. Abdulkarim, A. Mohanty, O. P. Acharya, Bhargav Appasani, Mohammad S. Khan, S. K. Mohapatra, F. F. Muhammadsharif, & J. Dong. (2022). A review on metamaterial absorbers: Microwave to optical. Frontiers in Physics, Sec. Optics and Photonics, 10, 1-18.
B.X. Wang, H. X. Zhu, & W. Q. Huang. (2019). Multiple-band ultra-thin perfect metamaterial absorber using analogy split-ring resonators. Plasmonics, 14, 1789–1800.
C.R. Williams, M. Misra, S. R. Andrews, S. A. Maier, S. Carretero-Palacios, S. G. Rodrigo, F. J. Garcia-Vidal, & L. Martin-Moreno. (2010). Dual band terahertz waveguiding on a planar metal surface patterned with annular holes. Appl. Phys. Lett., 96, 011101.
W.W. Salisbury. (1954). Absorbent body for electromagnetic waves. United States Patent 2599944.
G. Dayal, & S. A. Ramakrishna. (2013). Design of multi-band metamaterial perfect absorbers with stacked metal–dielectric disks. Journal of Optics, 15, 055106.
D. Yang, & Y. Xia. (2020). Experimental verification of multi-band metamaterial absorber with double structured layers. Materials Research Express, 7, 035801.
Y. He, B.X. Wang, P. Lou, & H. Zhu. (2020). Multiple-band absorber enabled by new type of metamaterial resonator formed by metallic split ring embedded with rectangle patch. Results in Physics, 18(103251), 1-5.
Y. J. Kim, J. S. Hwang, Y. J. Yoo, B. X. Khuyen, X. Chen, & Y. P. Lee. (2017). Triple-band metamaterial absorber based on single resonator. Current Applied Physics, 17, 1260-1263.
S. Ghosh, S. Bhattacharyya, D. Chaurasiya, & K. V. Srivastava. (2015). An ultrawideband ultrathin metamaterial absorber based on circular split rings. IEEE Antennas and Wireless Propagation Letters, 14, pp. 1172-1175.
S. Bhattacharyya, S. Ghosh, D. Chaurasiya, & K. V. Srivastava. (2015). Wide-angle broadband microwave metamaterial absorber with octave bandwidth. Aplied Physics A, 9, 1160–1166.
D. Sood, & C.C. Tripathi. (2015). A wideband ultrathin low profile metamaterial microwave absorber. Microwave & Optical Technology Letters, 57, 2723-2728.
D. Sood, & C.C. Tripathi. (2016). A wideband wide-angle ultrathin low profile metamaterial microwave absorber, 58, 1131-1135.
H. Zhai, C. Zhan, L. Liu, & Y. Zang. (2015). Reconfigurable wideband metamaterial absorber with wide angle and polarisation stability. Electronic Letters, 51, 1624-1626.
W. Li, T. Wu, W. Wang. J. Guan, & P. Zhai. (2014). Integrating non-planar metamaterials with magnetic absorbing materials to yield ultra-broadband microwave hybrid absorbers. Appl. Phys. Lett., 104, 022903.
Y. Shen, Z. Pei, Y. Pang, J. Wang, A. Zhang, & S. Qu. (2015). An extremely wideband and lightweight metamaterial absorber. Journal of Applied Physics, 117, 224503.
S. Ramya, I., & Srinivasa Rao. (2017). A compact ultra-thin ultra-wideband microwave metamaterial absorber. Microwave & Optical Technology Letters, 59, 1837-1845.
Y. Cheng, Y. Zou, H. Luo, F. Chen, & X. Mao. (2019). Compact ultra-thin seven-band microwave metamaterial absorber based on a single resonator structure. Journal of Electronic Materials, 48, 3939–3946.
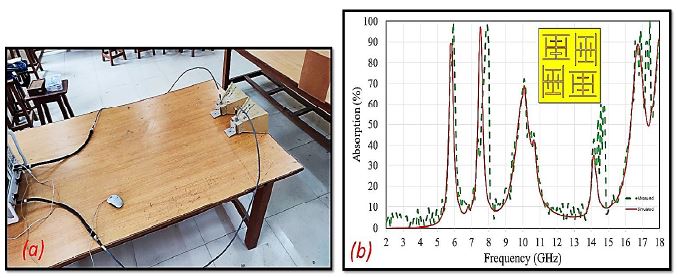
Published
How to Cite
Issue
Section
License
Copyright (c) 2022 Deepak Sood

This work is licensed under a Creative Commons Attribution 4.0 International License.